Methods for Quantifying, Projecting, and Managing the Health Risks of Climate Change
Abstract
A rapidly increasing literature base is quantifying associations between climate change and health outcomes. Here Ebi reviews methods for quantifying, projecting, and managing the health risks of climate change.
Introduction
Climate change is a stress multiplier, putting pressure on vulnerable systems, populations, and regions. Evidence-based research is critical to provide robust, useful, and useable insights at the geographic scale and level of detail most useful for decision-makers, to rapidly increase preparedness, and to incentivize immediate and widespread reductions in greenhouse gas (GHG) emissions.
It is unequivocal that humans are changing the planet, including the atmosphere, oceans, and biosphere,1 and that these changes are affecting population health and well-being.2,3 Human activities, particularly burning of fossil fuels and deforestation, are increasing the concentrations of GHGs in the atmosphere, which in turn are altering weather patterns worldwide.1 Carbon dioxide (CO2) is the main GHG of concern; other contributors include methane (e.g., from livestock, rice cultivation, and biomass burning) and nitrous oxide from nitrogen fertilizers. Particles also play important roles in altering warming, such as black carbon (increases warming) and sulfates (cooling effect). Overall, approximately 83% of all GHGs from fossil fuels were emitted during the lifetimes of people born in 1950. For those born in 1975, about 51% were emitted in their lifetime. For those born in 2000, the figure is about 28%.
Human activities added about 2500 billion tons of CO2 (GtCO2) to the atmosphere since 1850, of which the United States was responsible for 509 GtCO2 or some 20%. In 1950, the world emitted about 6 billion tons of CO2 per year.4 By 1990, annual emissions increased to more than 22 billion tons; annual emissions are now over 34 billion tons. China is the largest emitter currently; it is responsible for more than one quarter of current global emissions. Since 1971, over 1.5 trillion tons of CO2 were emitted, of which the United States was responsible for 25% and the European Union for 22%. In 2019, atmospheric CO2 concentrations were higher than at any time in at least 2 million years.4 Atmospheric CO2 was more than 419 ppm in February 20225; preindustrial concentrations were about 280 ppm.
These changes in atmospheric GHG concentrations affect the mean and variability of temperature and precipitation. Each of the last four decades was successively warmer than any previous decade since reliable records became available in the mid-1800s.1 The global mean surface temperature is now about 1.1°C above preindustrial temperatures, with larger increases over land (1.6°C) than oceans. Furthermore, because warmer air holds more water, these temperature changes are affecting the hydrologic cycle.1 The number of heavy rain events is increasing, resulting in more flooding in some regions. At the same time, droughts are increasing because warmer air draws more moisture from the soil. Overall, average precipitation over land likely increased since 1950.
Along with changes in the mean of temperature and precipitation, extremes are increasing, with heat waves, flooding, and drought becoming more frequent and intense. Heat waves over land that were 1-in 50-year events between 1850 and 1900 now occur 4.8 times more often.1 Such events are projected to occur 8.6 times more often with warming of 1.5°C and 13.9 times more often with warming of 2°C. Compound extreme events also are increasing, such as back-to-back heat waves, or heat waves and drought or wildfires; low soil moisture is contributing to wildfire risk.
The magnitude of future warming will depend on our GHG emission pathway.1 Immediate and extensive reductions in emissions, aiming for net-zero emissions by mid-century, could keep warming to under 2.0°C this century; mid-range emissions could result in warming of 2.1° to 3.5°C; and very high emissions could result in warming of 3.3° to 5.7°C this century. The last time the global surface temperature was at or greater than 2.5°C above preindustrial temperatures was over 3 million years ago.
Methods for Understanding Current and Future Health Risks of Climate Change
Quantifying current impacts, projecting future risks, and developing effective responses entail unique challenges. Challenges include that a reference period for health data has not been defined. Health risks constantly change over time, with changes in underlying health conditions (e.g., increases in chronic diseases), changes in exposures, and effectiveness of control programs. Furthermore, the extent of health data varies considerably across regions, with limited long-term data sets in many low- and middle-income countries.
Although everyone is exposed to a changing climate, exposures are not the same for everyone. Certain populations and regions are less resilient because of their location (e.g., coastal zones or areas prone to wildfires) or underlying poverty, inequities, and other vulnerabilities.6 At the same time, individuals, communities, and health systems are implementing changes, such as purchasing air conditioners or modifying vector control programs, to better manage increases in the geographic range and seasonality of diseases such as dengue fever or malaria.2
Ministries and departments of health typically start engaging with climate change and health by conducting a vulnerability and adaptation assessment to quantify and prioritize current and future health risks and to identify evidence-based interventions to increase health system resilience (Fig. 1). Such assessments go beyond addressing current vulnerabilities by identifying policies to protect health risks from future, and possibly more severe, climate change, including catastrophic events. The results provide the foundational information needed to develop local to national adaptation plans. These assessments are part of a risk management cycle that iteratively strengthens the core functions of health systems as the magnitude and pattern of climate-sensitive health outcomes evolve.7
Figure 1
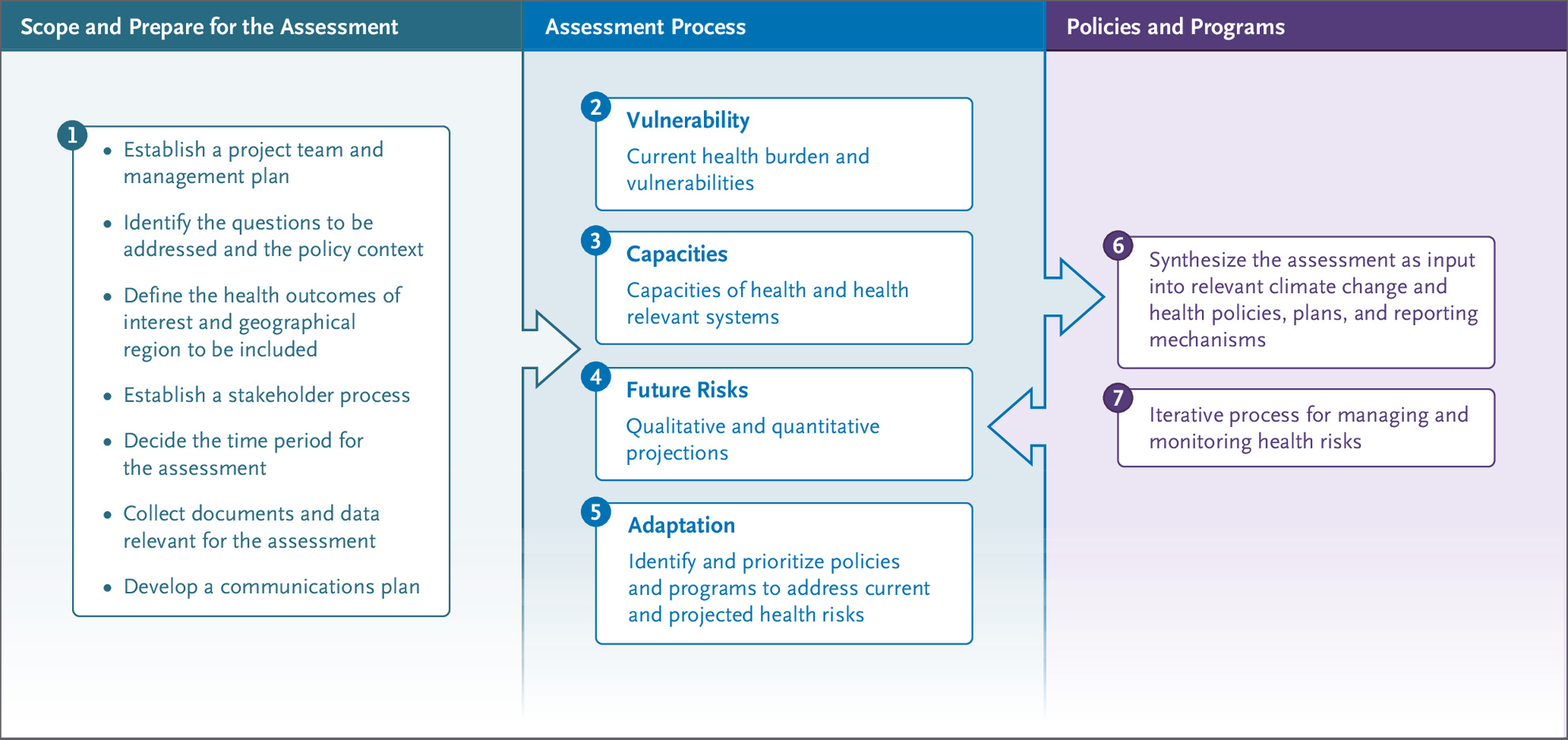
Research methods to provide policy-relevant information for a vulnerability and adaptation assessment are generally based on standard health impact methods; others are adapted to account for the unique issues with studying weather and climate as exposure variables. Methods include the following:
•
Quantify the magnitude and burden of climate-sensitive health outcomes;
•
Estimate the extent to which climate change is already causing illness, injuries, and deaths;
•
Project the magnitude and pattern of health risks under a range of climate and development scenarios;
•
Develop, deploy, monitor, and evaluate the effectiveness of policies and programs to increase health system resilience;
•
Estimate the costs to individuals and health systems of the health risks of climate variability and change; and
•
Estimate the health cobenefits of mitigation policies and technologies.
Quantify the Magnitude and Burden of Climate-Sensitive Health Outcomes
A rapidly increasing literature base is quantifying associations between weather and climate patterns and climate-sensitive health outcomes. In North America, between January 1, 2013 and February 14, 2020, the most studied health outcomes were heat-related morbidity and mortality (35.9%), respiratory health outcomes (25.1%), and general causes of morbidity and mortality (24.2%).8 Temperature was the most common weather variable investigated (76.2%), followed by precipitation (30.2%). Heat events were the most common climate hazard (32.8%), followed by changes in air quality (30.0%). Other climate-sensitive health outcomes were much less studied.
Identifying weather variables of interest should be based on understanding of disease etiology (particularly for infectious diseases). Epidemiology studies show that exposure to high ambient temperatures increases morbidity and mortality, reduces worker productivity, increases adverse pregnancy outcomes, and negatively affects mental health.9,10 For example, self-reported mental health in the United States is improved on cooler days, whereas higher temperatures increase the probability of reporting poor mental health.11 Daily or weekly associations between temperature or precipitation and health outcomes are increasingly described using distributed lag nonlinear models to estimate trends over time, accounting for potential lags between exposure and health responses.12 For example, the role of temperature in seasonal variations in mortality varies across cities and countries in tropical, dry, temperate, and continental climate zones, with the highest impacts in temperate and continental climate zones.13 Lags vary by health outcome, with a lag of about 24 hours between the start of a heat wave and increased mortality14; the lag between heavy precipitation events and increased diarrheal disease is several weeks.15
Research and practice have identified populations and regions particularly vulnerable to changing weather patterns.2 A helpful tool for decision-makers is mapping hotspots of vulnerability and projecting how these could change over time as climate-sensitive health outcomes shift their geographic range, to help communicate where impacts are most likely to occur and to prioritize actions.16 Mapping vulnerabilities should be done at the finest geographic scale possible, to facilitate community understanding of the current challenges associated with climate change and how those could alter over time. However, these analyses are often hindered by the difficulty in obtaining fine-scale health outcome data due to laws intended to ensure confidentiality of these data.
Estimate the Extent to Which Climate Change Is Already Causing Illness to Be Parallel with Injuries and Deaths
Statistical methods developed by climate scientists, termed detection and attribution, are quantifying the extent to which a single event or a longer-term trend could be attributed to climate change.17 Models or observations are used to determine whether a system has changed, such as the number or intensity of heat waves, or the number of hospitalizations and deaths in a heat wave.18 When a change is detected, the extent to which climate change could have caused that change is quantified. Limited detection and attribution studies for health impacts have mostly focused on heat wave–related mortality. An example is the heat dome that settled over the U.S. Pacific Northwest and western Canada at the end of June 2021.19 A detection and attribution study determined that the heat wave intensity was virtually impossible in the absence of anthropogenic climate change. The heat wave caused more than 1000 excess human deaths and a 69-fold increase in heat-related emergency department visits compared with the same period in 2019; other critical infrastructure was affected. In some coastal areas, high intertidal temperatures combined with low tides resulted in the deaths of over a million shellfish, affecting livelihoods, food security, and recreation. A modeling study estimated that 37% of heat-related deaths in 43 countries over recent decades were caused by climate change.20
Project the Magnitude and Pattern of Health Risks under a Range of Climate and Development Scenarios
The health risks of climate change result from the intersection of the hazards created by changing weather patterns, the extent to which populations are exposed to those hazards, the vulnerability of those so exposed, and the capacity of individuals and health systems to prepare for and manage changing health risks.21 Quantitative methods can be used to estimate relationships and extrapolate future burdens and risks. Expert judgment and development of qualitative scenarios also can be used by health professionals to estimate future impacts.7 Qualitative projections consider recent trends in weather variables, extreme weather events, and/or sea level rise; trends in the burden of climate-sensitive health outcomes (e.g., in cases of dengue fever); and planned modifications to policies and programs (e.g., increasing resources for the dengue control program). Expert judgment is used to combine these sources of information into statements about the extent to which climate change could alter disease burdens over coming decades.22
Quantitative projections of health risks use exposure-response relationships, generally based on short-term associations between weather variables and health outcomes, and projected changes in weather and climate trends (or extremes) to estimate changes over time in the burden of disease of interest. Two basic types of models are used: empirical (statistical) and process-based (biological or mechanistic), often reaching different conclusions. Empirical (statistical) models use observations to establish relationships between a health outcome and one or more weather and non-meteorological (e.g., socioeconomic or demographic) variables.23 Projected changes in weather variables are used to estimate the future distribution or burden of a health outcome, generally assuming linear relationships. Challenges include data availability, accuracy, and length of time series. For vector-borne disease models, observations of current disease patterns reflect the effectiveness of control programs and other variables. Because validated absence data are often not available, these models may not accurately reflect the environmental suitability of a location for transmission.
Process-based models are built from equations describing the dynamics of disease etiology; they generally simulate the impact of weather variables on the health outcomes of interest using equations describing disease burdens at daily or weekly time steps.24 Projected changes in weather variables under different climate change scenarios are used to estimate the future distribution and burden of the health outcome. Challenges to process-based modeling include poorly constrained values for parameters in the equations, the assumption of a causal relationship, and the possibility of unknown processes not described in the model.
Because the current magnitude and pattern of health burdens is at least partially the result of past investments in health systems and development choices such as urbanization plans, the degree to which current exposure-response relationships are predictive of future patterns of climate-sensitive ill health is unclear.25 Projecting risks requires using scenarios that describe not just temperature change over this century, but also the evolution of the social and environmental determinants of health, specific adaptation policies, and other factors that can influence the incidence and geographical range of climate-sensitive health outcomes.24
Develop, Deploy, Monitor, and Evaluate the Effectiveness of Policies and Programs to Increase Health System Resilience
Climate-sensitive health outcomes are among the leading causes globally of current morbidity and mortality, particularly for children.2 Health ministries and departments and other health organizations have a wide range of options to manage these outcomes, although few policies and programs explicitly incorporate climate change. Therefore, health systems may not be adequately prepared for climate-related shocks and stresses. Proactive assessment of the effectiveness, strengths, and weaknesses of these programs can provide critical insights for identifying possible modifications to increase the capacity to manage the additional health burden due to climate change.7
Assessments of the capacity of health systems to deal with climate-related shocks and stresses focus on the required capacities for each building block of climate-resilient and environmentally sustainable health systems, as outlined in the World Health Organization operational framework (Fig. 2).26 The capacity assessment should include specific climate change effects (e.g., floods), the specific health outcomes of interest (e.g., diarrheal diseases), and broader impacts (e.g., disruption of water and energy access in health care facilities). For example, reducing the burden of temperature-sensitive waterborne diseases requires improved access to safe water and improved sanitation, in addition to explicitly including climate change into health system policies and programs.
Figure 2
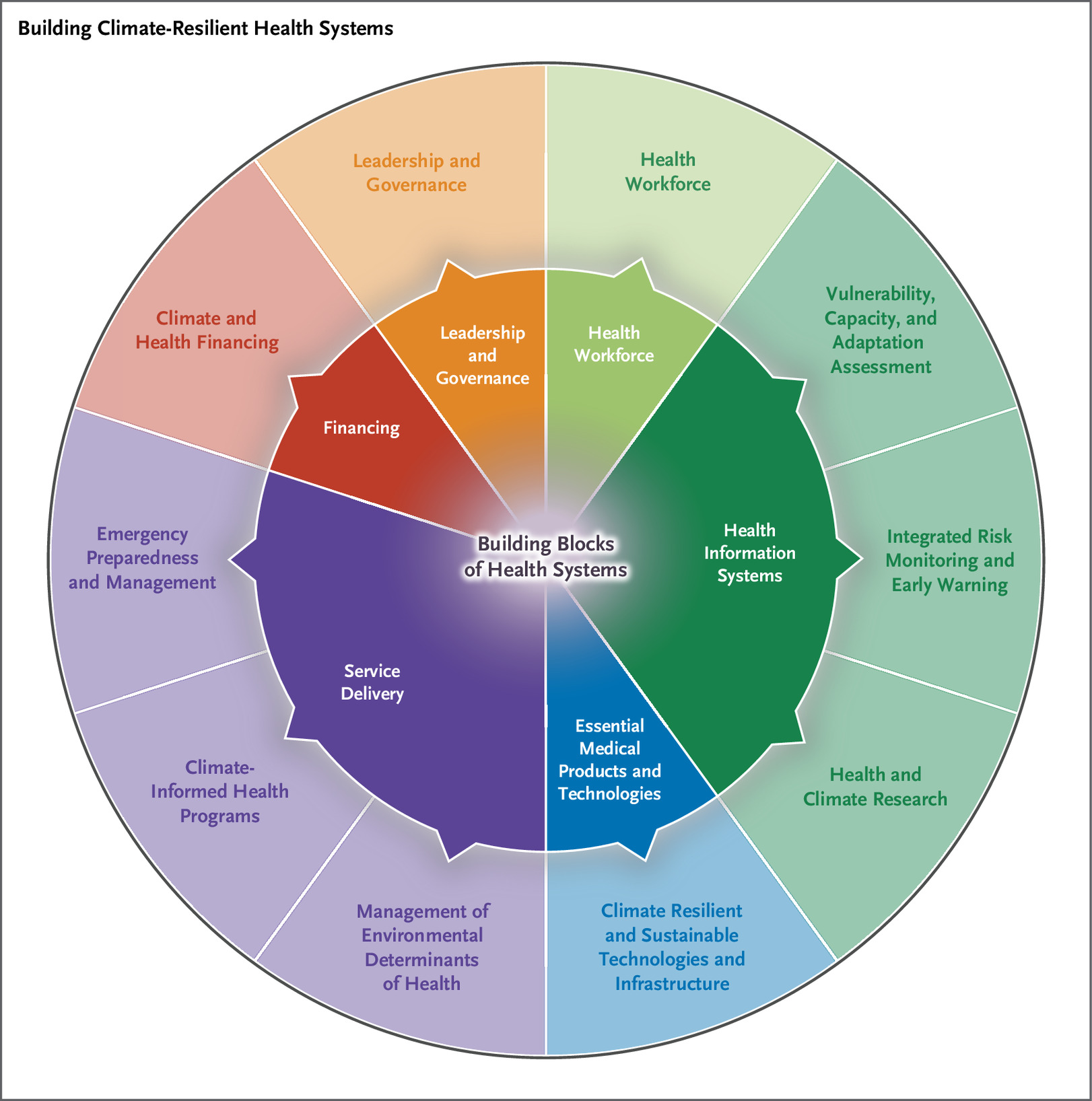
Within a health system, a range of organizations have individual or joint responsibility for managing climate-sensitive health outcomes, including the ministry or department of health, civil organizations, private-sector actors, and others.7 For example, health departments typically have responsibility for vector-borne disease surveillance and control programs, while disaster risk reduction activities may be joint across health, emergency management, and other departments and may include the Red Cross or other organizations. A capacity assessment should consult with all relevant organizations and institutions to determine what is working well, what could be improved, and the capacity of the programs to address possible increases in the incidence or changes in seasonality or geographical range of the health outcomes of concern.
This information can then be used to identify opportunities for increasing resilience by, for example, improving monitoring and surveillance, developing and deploying early warning and response systems, augmenting education and training for health care providers and the public, modifying regulations for water- and foodborne diseases to account for projected changes in access to clean water, and improving emergency response plans.7 These changes should be structured such that their effectiveness can be evaluated in a continuous cycle of learning.
Estimate the Costs to Individuals and Health Systems of the Health Risks of Climate Variability and Change
Comprehensive estimates of the costs and benefits of actions to reduce the health risks of climate change include the following: costs for health systems to respond to a climate-related event; the costs of injuries, illnesses, and deaths and of lost work time or reduced productivity; the costs of increasing the resilience of health systems to future climate change; the costs associated with failures to adapt to or mitigate the risks of climate change; and the costs of managing residual risks, acknowledging that health systems are unlikely to be fully resilient to increasing impacts of climate change. These estimates should include resource costs (e.g., medical care costs), opportunity costs (e.g., lost productivity), and welfare costs (e.g., pain and suffering).23,27
For example, the health-related costs from 10 climate-sensitive events, including wildfires, high ozone, infectious disease outbreaks, pollen, harmful algal blooms, and extreme weather, in 11 U.S. states in 2012 were estimated at $10.0 billion from 917 deaths, 20,568 hospitalizations, and 17,857 emergency department visits.28 Between 2015 and 2019, the economic impacts of the health effects from heat waves in France were €25.5 billion, from mortality (€23.2 billion), minor restricted activity days (€2.3 billion), and morbidity (€0.3 billion).29
Future health care costs from exposure to extreme heat in 2041 to 2070 in Michigan were projected to be $280 million for nonaccidental mortality and $14 million for heat-associated emergency department visits.30 Better quantification of these significant health costs can justify investments in adaptation and mitigation.
Estimate the Health Cobenefits of Mitigation Policies and Technologies
International agreements and national and state mitigation actions are aiming to rapidly decrease GHG emissions to reduce the magnitude and destructiveness of future climate variability and change. Economic valuations of the associated avoided hospitalizations and premature deaths indicate that they generally offset a significant proportion, if not all, of the costs of implementing mitigation policies, providing further incentives for aggressive policy action. That is, mitigation policies should be implemented because of the significant improvements to population health in addition to the benefits for achieving climate policy. Further estimates would help policy makers prioritize investments based on mitigation potential and expected health benefits.
Mitigation policies benefit health through the following: reducing exposure to air pollutants from coal-fired power plants and other stationary sources, increasing physical activity from walking and biking, and encouraging dietary changes that increase consumption of whole grains, vegetables, and fruit over red meat.31 Benefits from these policies accrue quickly and locally. Examples include $350 million in benefits to child health over the period from 2009 to 2014 from the U.S. Regional Greenhouse Gas Initiative.32 Another example is the projected health benefits of mitigation policies in energy, food and agriculture, and transport sectors across nine countries in 2040.33 Benefits were attributable to the mitigation of direct GHG emissions and the commensurate actions that reduce exposure to harmful pollutants as well as to improved diets and safe physical activity. Compared with the current pathway, a sustainable pathway consistent with the goal of the Paris Agreement and the Sustainable Development Goals projected an annual reduction of 1.18 million air pollution–related deaths, 5.86 million diet-related deaths, and 1.15 million deaths due to physical inactivity. Adopting a more ambitious health-in-all climate policies scenario could result in a further reduction of 462,000 annual deaths attributable to air pollution, 572,000 annual deaths attributable to diet, and 943,000 annual deaths attributable to physical inactivity.
To strengthen the policy relevance of health cobenefits research, an expert panel of researchers developed recommendations for conducting and reporting estimates, including the following: through harmonization of approaches for stakeholder engagement, modeling inputs and assumptions, parameterization and reporting, approaches to uncertainty and sensitivity analysis, accounting for policy uptake, and discounting.31
About 8.3% of the U.S. GHG emissions are from health care, indicating significant scope for greening the health sector through increasing energy efficiency of health care facilities, using renewable energy, and reducing emissions throughout the supply chain, particularly when GHG emissions are not correlated with health systems quality.34 The estimated disease burden in 2018 from these emissions was about 388,000 disability-adjusted life years; this is similar to the years of lives lost from preventable medical errors.
Conclusions
The climate change and health research agenda laid out over 30 years ago35 remains valid, with wide-ranging opportunities to apply current methods and develop new approaches to generate the needed insights to increase the resilience of communities and health systems in the context of an unstable climate. Better understanding of current and future risks, and iterative risk management options, is urgently needed to rapidly increase preparedness and incentivize immediate and widespread reductions in GHG emissions.
Notes
Disclosure forms provided by the authors are available with the full text of this article.
Supplementary Material
Disclosure Forms (evidra2200002_disclosures.pdf)
- Download
- 84.10 KB
References
1.
Intergovernmental Panel on Climate Change. Summary for policymakers. In: Masson-Delmotte V, Zhai P, Pirani A, et al., eds. Climate change 2021: the physical science basis. Cambridge, UK: Cambridge University Press, 2021:3-32.
2.
Cisse G, McLeman R, Adams H, et al. Chapter 7: Health, wellbeing, and the changing structure of communities. In: Portner H-O, Roberts DC, Tignor M, et al. Climate change 2022: impacts, adaptation and vulnerability. Cambridge, UK: Cambridge University Press, 2022:9-181.
3.
Romanello M, McGushin A, Di Napoli C, et al. The 2021 report of the Lancet countdown on health and climate change: code red for a healthy future. Lancet 2021;398:1619-1662.
4.
Ritchie H, Roser M. CO2 and other greenhouse gas emissions. 2019 (https://ourworldindata.org/greenhouse-gas-emissions).
5.
National Oceanic and Atmospheric Administration. Global monitoring laboratory: carbon cycle greenhouse gases. 2021 (https://gml.noaa.gov/ccgg/trends/).
6.
Allen MR, Babiker M, Chen Y, et al. Summary for policymakers. In: Masson-Delmotte V, Zhai P, Portner HO, et al., eds. Global warming of 1.5°C. Cambridge, UK: Cambridge University Press, 2018:3-24.
7.
World Health Organization. Climate change and health: vulnerability and adaptation assessment. 2021 (https://www.who.int/publications/i/item/9789240036383).
8.
Harper SL, Cunsolo A, Babujee A, et al. Trends and gaps in climate change and health research in North America. Environ Res 2021;199:111205.
9.
Haines A, Ebi K. The imperative for climate action to protect health. N Engl J Med 2019;380:263-273.
10.
Ebi KL, Boyer C, Ogden N, et al. Burning embers: synthesis of the health risks of climate change. Environ Res Lett 2021;16:044042.
11.
Li M, Ferreira S, Smith TA. Temperature and self-reported mental health in the United States. PLoS One 2020;15:e0230316.
12.
Gasparrini A. Correction to “Modeling exposure-lag-response associations with distributed lag non-linear models”. Stat Med 2014;33:900.
13.
Madaniyazi L, Armstrong B, Chung Y, et al. Seasonal variation in mortality and the role of temperature: a multi-country multi-city study. Int J Epidemiol 2022;51:122-133.
14.
Ebi KL, Capon A, Berry P, et al. Hot weather and heat extremes: health risks. Lancet 2021;398:698-708.
15.
Levy K, Smith SM, Carlton EJ. Climate change impacts on waterborne diseases: moving toward designing interventions. Curr Environ Health Rep 2018;5:272-282.
16.
Harrigan RJ, Thomassen HA, Buermann W, Smith TB. A continental risk assessment of West Nile virus under climate change. Glob Change Biol 2014;20:2417-2425.
17.
Otto FEL, Harrington L, Schmitt K, et al. Challenges to understanding extreme weather changes in lower income countries. Bull Am Meteorol Soc 2020;101:E1851-E1860.
18.
Ebi KL, Åström C, Boyer CJ, et al. Using detection and attribution to quantify how climate change is affecting health. Health Aff (Millwood) 2020;39:2168-2174.
19.
Philip SY, Kew SF, van Oldenborgh GJ, et al. Rapid attribution analysis of the extraordinary heatwave on the Pacific Coast of the US and Canada June 2021. November 21, 2021 (https://esd.copernicus.org/preprints/esd-2021-90/).
20.
Vicedo-Cabrera AM, Scovronick N, Sera F, et al. The burden of heat-related mortality attributable to recent human-induced climate change. Nat Clim Chang 2021;11:492-500.
21.
Intergovernmental Panel on Climate Change. Summary for policymakers. In: Field CB, Barros V, Stocker D, eds. Managing the risks of extreme events and disasters to advance climate change adaptation. Cambridge, UK: Cambridge University Press, 2012:3-24.
22.
Lindgren E, Andersson Y, Suk JE, Sudre B, Semenza JC. Public health: monitoring EU emerging infectious disease risk due to climate change. Science 2012;336:418-419.
23.
Ebi KL, Hondula D, Kinney P, et al. Health risks of climate variability and change. In: Gelfand AE, Fuentes M, Hoeting JA, Smith RL, eds. Handbook of environmental and ecological statistics. Boca Raton, FL: CRC Press, 2019:819-839.
24.
Ebi KL, Hess JJ, Watkiss P. Health risks and costs of climate variability and change. In: Mock CN, Nugent R, Kobusingye O, Smith KR, eds. Disease control priorities, 3rd ed. Washington, DC: World Bank, 2017:153-169.
25.
Rocklöv J, Ebi K. High dose extrapolation in climate change projections of heat-related mortality. J Agric Biol Environ Stat 2012;17:461-475.
26.
World Health Organization. Operational framework for building climate resilient health systems. Geneva, Switzerland: WHO, 2015.
27.
Limaye VS, Max W, Constible J, Knowlton K. Estimating the costs of inaction and the economic benefits of addressing the health harms of climate change. Health Aff (Millwood) 2020;39:2098-2104.
28.
Limaye VS, Max W, Constible J, Knowlton K. Estimating the health-related costs of 10 climate-sensitive U.S. events during 2012. Geohealth 2019;3:245-265.
29.
Adélaïde L, Chanel O, Pascal M. Health effects from heat waves in France: an economic evaluation. Eur J Health Econ 2022;23:119-131.
30.
Gronlund CJ, Cameron L, Shea C, O’Neill MS. Assessing the magnitude and uncertainties of the burden of selected diseases attributable to extreme heat and extreme precipitation under a climate change scenario in Michigan for the period 2041–2070. Environ Health 2019;18:40.
31.
Hess JJ, Ranadive N, Boyer C, et al. Guidelines for modeling and reporting health effects of climate change mitigation actions. Environ Health Perspect 2020;128:115001.
32.
Perera F, Cooley D, Berberian A, Mills D, Kinney P. Co-benefits to children’s health of the U.S. Regional Greenhouse Gas Initiative. Environ Health Perspect 2020;128:77006.
33.
Hamilton I, Kennard H, McGushin A, et al. The public health implications of the Paris Agreement: a modelling study. Lancet Planet Health 2021;5:e74-e83.
34.
Eckelman MJ, Huang K, Lagasse R, Senay E, Dubrow R, Sherman JD. Health care pollution and public health damage in the united states: an update. Health Aff (Millwood) 2020;39:2071-2079.
35.
McMichael AJ, McMichael T. Planetary overload: global environmental change and the health of the human species. Cambridge, UK: Cambridge University Press, 1993.
Information & Authors
Information
Published In
NEJM Evidence
Editor
C. Corey Hardin, M.D., Ph.D., Editor
Copyright
Copyright © 2022 Massachusetts Medical Society.
History
Published online: July 26, 2022
Published in issue: July 26, 2022
Topics
Authors
Metrics & Citations
Metrics
Altmetrics
Citations
Export citation
Select the format you want to export the citation of this publication.
Cited by
- Addressing Capacity Constraints of Rural Local Health Departments to Support Climate Change Adaptation: Action Is Needed Now, International Journal of Environmental Research and Public Health, 19, 20, (13651), (2022).https://doi.org/10.3390/ijerph192013651
Loading...